That reactor, a design called the RBMK-1000, was discovered to be fundamentally flawed after the Chernobyl accident.
And yet there are still 10 of the same type of reactor in operation in Russia. How do we know if they’re safe?
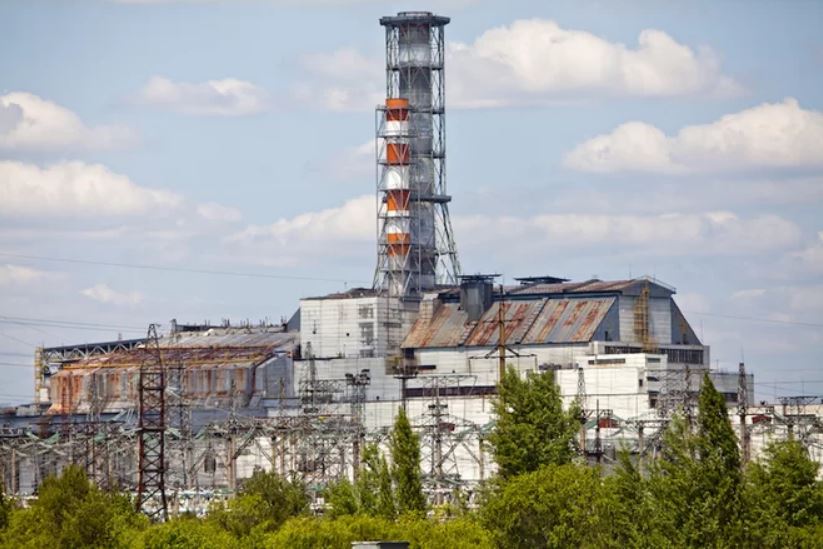
The short answer is, we don’t. These reactors have been modified to lessen the risk of another Chernobyl-style disaster, experts say, but they still aren’t as safe as most Western-style reactors. And there are no international safeguards that would prevent the construction of new plants with similar flaws.
“There are a whole number of different types of reactors that are being considered now in various countries that are significantly different from the standard light-water reactor, and many of them have safety flaws that the designers are downplaying,” said Edwin Lyman, a senior scientist and the acting director of the Nuclear Safety Project at the Union of Concerned Scientists.
“The more things change, the more they stay the same,” Lyman said.
Reactor 4
At the center of the Chernobyl disaster was the RBMK-1000 reactor, a design used only in the Soviet Union. The reactor was different from most light-water nuclear reactors, the standard design used in most Western nations.
Light-water reactors consist of a large pressure vessel containing nuclear material (the core), which is cooled by a circulating supply of water. In nuclear fission, an atom (uranium, in this case), splits, creating heat and free neutrons, which zing into other atoms, causing them to split and release heat and more neutrons. The heat turns the circulating water to steam, which then turns a turbine, generating electricity.
In light-water reactors, the water also acts as a moderator to help control the ongoing nuclear fission within the core. A moderator slows down free neurons so that they’re more likely to continue the fission reaction, making the reaction more efficient. When the reactor heats up, more water turns to steam, and less is available to play this moderator role. As a result, the fission reaction slows. That negative feedback loop is a key safety feature that helps keep the reactors from overheating.
The RBMK-1000 is different. It also used water as a coolant, but with graphite blocks as the moderator. The variations in the reactor design allowed it to use less-enriched fuel than usual and to be refueled while running. But with the coolant and moderator roles separated, the negative feedback loop of “more steam, less reactivity,” was broken. Instead, RBMK reactors have what’s called a “positive void coefficient.”
When a reactor has a positive void coefficient, the fission reaction speeds up as the coolant water turns to steam, rather than slowing down. That’s because boiling opens up bubbles, or voids, in the water, making it easier for neutrons to travel right to the fission-enhancing graphite moderator, said Lars-Erik De Geer, a nuclear physicist who is retired from the Swedish Defence Research Agency.
From there, the problem builds: The fission becomes more efficient, the reactor gets hotter, the water gets steamier, the fission becomes more efficient still, and the process continues.
Run-up to disaster
When the Chernobyl plant was running at full power, this wasn’t a big problem, Lyman said. At high temperatures, the uranium fuel that powers the fission reaction tends to absorb more neutrons, making it less reactive.
At low power, though, RBMK-1000 reactors become very unstable. In the run-up to the Chernobyl accident on April 26, 1986, operators were doing a test to see if the plant’s turbine could run emergency equipment during a power outage. This test required running the plant at reduced power. While the power was lowered, the operators were ordered by Kiev’s power authorities to pause the process. A conventional plant had gone offline, and Chernobyl’s power generation was needed.
“That was very much the main reason why it all happened in the end,” De Geer said.
The plant ran at partial power for 9 hours. When the operators got the go-ahead to power most of the rest of the way down, there had been a buildup of neutron-absorbing xenon in the reactor, and they couldn’t maintain the appropriate level of fission. The power fell to nearly nothing. Trying to boost it, the operators removed all of the control rods, which are made of neutron-absorbing boron carbide and are used to slow the fission reaction. Operators also reduced the flow of water through the reactor. This exacerbated the positive void coefficient problem, according to the Nuclear Energy Agency. Suddenly, the reaction became very intense indeed. Within seconds, the power surged to 100 times what the reactor was designed to withstand.
There were other design flaws that made it difficult to get the situation back under control once it started. For example, the control rods were tipped with graphite, De Geer says. When the operators saw that the reactor was starting to go haywire and tried to lower the control rods, they got stuck. The immediate effect was not to slow the fission, but to enhance it locally, because the additional graphite at the tips initially boosted the fission reaction’s efficiency nearby.
Two explosions rapidly followed. Scientists still debate exactly what caused each explosion. They both may have been steam explosions from the rapid increase in pressure in the circulation system, or one may have been steam and the second a hydrogen explosion caused by chemical reactions in the failing reactor. Based on the detection of xenon isotopes at Cherepovets, 230 miles (370 kilometers) north of Moscow after the explosion, De Geer believes that the first explosion was actually a jet of nuclear gas that shot several kilometers into the atmosphere.
Changes made
The immediate aftermath of the accident was “a very unnerving time” in the Soviet Union, said Jonathan Coopersmith, a historian of technology at Texas A&M University who was in Moscow in 1986. At first, the Soviet authorities kept information close; the state-run press buried the story, and the rumor mill took over. But far away in Sweden, De Geer and his fellow scientists were already detecting unusual radioactive isotopes. The international community would soon know the truth.
On May 14, Soviet leader Mikhail Gorbachev gave a televised speech in which he opened up about what had happened. It was a turning point in Soviet history. “It made glasnost real,” Coopersmith said, referring to the nascent policy of transparency in the Soviet Union.
It also opened a new era in cooperation for nuclear safety. In August 1986, the International Atomic Energy Agency held a post-accident summit in Vienna, and Soviet scientists approached it with an unprecedented sense of openness, said De Geer, who attended. “It was amazing how much they told us,” he said.
Among the changes in response to Chernobyl were modifications to the other RBMK-1000 reactors in operation, 17 at the time. According to the World Nuclear Association, which promotes nuclear power, these changes included the addition of inhibitors to the core to prevent runaway reactions at low power, an increase in the number of control rods used in operation and an increase in fuel enrichment. The control rods were also retrofitted so that the graphite would not move into a position that would increase reactivity.
Chernobyl’s other three reactors operated till 2000 but have since closed, as have two more RBMKs in Lithuania, which were shut down as a requirement of that country entering the European Union. There are four RBMK reactors operating in Kursk, three in Smolensk and three in St. Petersburg.
These reactors “aren’t as good as ours,” De Geer said, “but they are better than they used to be.“
“There were fundamental aspects of the design that couldn’t be fixed no matter what they did,” Lyman said. “I would not say they were able to increase the safety of the RBMK overall to the standard you’d expect from a Western-style light water reactor.“
In addition, De Geer pointed out, the reactors weren’t built with full containment systems as seen in Western-style reactors. Containment systems are shields made of lead or steel meant to contain radioactive gas or steam from escaping into the atmosphere in the event of an accident.
Oversight overlooked?
Despite the potentially international effects of a nuclear plant accident, there is no binding international agreement on what constitutes a “safe” plant, Lyman said.
The Convention on Nuclear Safety requires countries to be transparent about their safety measures and allows for peer review of plants, he said, but there are no enforcement mechanisms or sanctions. Individual countries have their own regulatory agencies, which are only as independent as local governments enable them to be, Lyman said.
“In countries where there is rampant corruption and lack of good governance, how can you expect that any independent regulatory agency is going to be able to function?” Lyman said.
Though no one besides the Soviet Union made RBMK-1000 reactors, some proposed new reactor designs do involve a positive void coefficient, Lyman said. For example, fast-breeder reactors, which are reactors that generate more fissile material as they generate power, have a positive void coefficient. Russia, China, India and Japan have all built such reactors, though Japan’s is not operational and is planned for decommission and India’s is 10 years behind schedule for opening.
“The designers are arguing that if you take everything into account, overall they’re safe, so that doesn’t matter that much,” Lyman said. “But designers shouldn’t be overconfident in their systems,” he said.
“That kind of thinking is what got the Soviets into trouble,” he said. “And it’s what can get us into trouble, by not respecting what we don’t know.“
Now we have to live with this terrifying unknown. Which of these 10 Chernobyl-style reactors still operating in Russia is going to explode next?